Unburning CO₂: The Problem with Fossil Carbon Capture and Utilization
Technologies that turn carbon dioxide into fuels or chemical resources can play a role in a climate-neutral future. But they come with challenges and usually only delay emissions. This can lead to overestimating their potential.
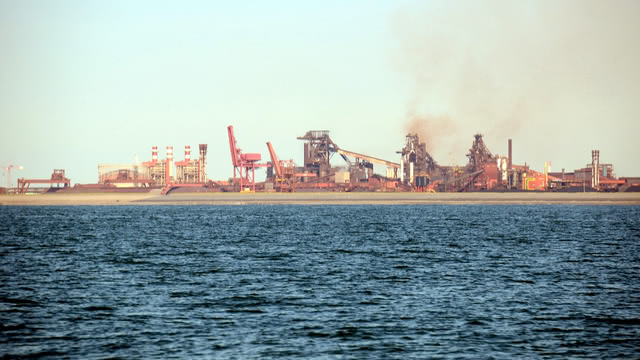
It sounds good: Instead of emitting carbon dioxide into the atmosphere, we could use it to make useful products. It is the key idea behind a set of technologies known as carbon capture and utilization - or short CCU. But while these technologies are promising, implementing them at scale comes with considerable challenges, and the common narratives around CCU lead to misunderstandings and overestimates of emissions savings.
The term CCU is not used consistently, so it is best to clarify what we are talking about. Carbon dioxide has some uses in food production, such as increasing yields in greenhouses or making sparkling drinks. Also, a special case is using CO₂ in enhanced oil recovery.
For this text, when talking about CCU, we are not talking about these direct uses of CO₂. Instead, we are talking about turning CO₂ into other carbon-containing chemicals.
Turning Hydrogen and CO₂ into Carbon-based Chemicals
These technologies often start with making hydrogen. Today, almost all hydrogen is made from fossil fuels, and hydrogen production is a substantial source of emissions by itself. It can only become a climate solution if it is produced in different ways, for example, by using renewable electricity to split water into hydrogen and oxygen, a process called electrolysis.
In further steps, hydrogen and carbon dioxide can be turned into substances currently made from fossil sources. It is effectively reversing the process of burning fossil fuels. Many CCU projects target making methanol (CH₃OH), the simplest carbon-based liquid chemical. Such green methanol could be directly used as a fuel or converted into other fuels or basic chemicals.
CCU technologies could help to avoid emissions in sectors where conventional decarbonization technologies hit their limits. In some applications, using renewable electricity directly is impossible, as neither batteries nor hydrogen can provide the required energy density. Notably, this is true for long-distance shipping and aviation. This is why e-fuels - another word for CCU-based fuels - are proposed as a solution in these sectors.
Ships can use methanol directly as a fuel. Methanol's energy density is still too low for planes, but CCU-based technologies could also produce kerosene. It can serve as a direct replacement for conventional jet fuel.
Another possible use case of CCU technologies is the production of basic chemicals used to make products. Methanol can be converted into ethylene and propylene, the basic building blocks of plastics. Alternatively, a Fischer-Tropsch process can provide naphtha. (See also: How to make Plastics without Fossil Fuels.)
Despite sounding attractive at first glance, CCU technologies come with considerable challenges and costs. But for fossil-based chemicals and energy-dense fuels, alternatives in a fossil-free world are limited. Apart from reducing use and increasing efficiency, the only other option is biomass.
Replacing fossil fuels with cultivated plants often has very high lifecycle emissions. Climate-friendly biomass sources like waste from food production or wood processing are only available in limited quantities.
Why saying CCUS is misleading
CCU is often mentioned alongside carbon capture and storage (CCS), and both are commonly referred to as CCUS (carbon capture utilization and storage). This is misleading. While both technologies start with the same CO₂ capture technologies, they have very different roles in a decarbonized economy.
Carbon capture and storage is the idea of capturing CO₂ from an emission source and storing it permanently underground. That comes with its own challenges and limitations. (CCS and some of its problems were covered in a previous newsletter.)
The ultimate goal of CCS is to store CO₂ permanently. That is very different from CCU, where, in most cases, carbon is only stored temporarily.
In the case of e-fuels, this is rather obvious: Let's say we take CO₂ from an industrial plant powered by fossil fuels. If we turn that CO₂ into jet fuel and burn it in a plane engine, we release CO₂ into the atmosphere. If the industrial plant claims to have avoided its CO₂ emissions, and the e-fuels producer sells them as carbon neutral, emission reductions are counted twice.
Such a process chain can lead to lower emissions than a comparable scenario where industrial emissions are directly released into the atmosphere, and the plane is powered by fossil kerosene. But even in almost ideal conditions and with no CO₂ leakage, this can never avoid emissions entirely. It has an upper limit of 50 percent emissions savings when looking at the combined emissions. (Theoretically, some additional savings are possible if we consider avoided upstream fossil fuel emissions.)
A good way of looking at such a process chain is that CCU with a fossil CO₂ source delays and reduces emissions - but it cannot avoid them.
What about products like plastics? In this case, carbon molecules are stored in the product. But we also need to consider end-of-life emissions. When plastics are burned in waste incinerators, they release all their carbon content as CO₂. Plastics in nature or landfills can degrade to highly potent greenhouse gases like methane or ethylene. It can usually be assumed that most of the carbon content of plastics and other chemical products will eventually end up in the atmosphere. Recycling only adds additional loops of carbon molecule use.
The only scenario in which plastics or other products can be considered as permanent carbon storage is if the carbon is permanently bound at their end of life. Theoretically, plastics can effectively be buried in properly managed landfills, and some argue that we should landfill end-of-life plastics. But going back to landfills at scale is often not an option due to space requirements. It may be an option in countries with a low population density. Another possibility is to equip waste incinerators with CCS, which is also not easy.
Potential CCU technologies that could avoid emissions permanently are ones that bind carbon in minerals. While interesting for some niche use cases, even that comes with caveats. Often, the source materials of those processes are materials that have released carbon earlier that gets re-absorbed. (Examples include carbon curing in cement production or remineralization of cement waste.)
CCU projects can have a different role and a place in a climate-neutral economy if they use different sources of CO₂, either biogenic or from direct air capture.
Plants use CO₂ from the air when they grow. Therefore, CO₂ released from plant material usually comes from the atmosphere. Thus, it is often claimed that biofuels or bio-based materials are climate-neutral.
But that view is too simplistic. While biogenic carbon itself is not from fossil sources, producing those biogenic sources involves emissions - from land use changes, fertilizers, or the production process. These upstream emissions should not be ignored. Still, using carbon dioxide from climate-friendly biomass sources is probably an important path forward for CCU.
It is also possible to supply CO₂ directly from the atmosphere. Such direct air capture technology, or DAC, has gained much attention in recent years, but the technology is still in its early stages. Costs are uncertain and likely high, as the atmosphere contains only around 0,04 percent CO₂.
Relying on Fossil Emissions risks dangerous Lock-In
Energy company Engie and Infinium, a US-based e-fuels startup, have announced plans to build an e-fuels facility called Reuze at the port of Dunkirk in France.
They plan to use CO₂ from ArcelorMittal's steelmaking facilities to produce e-fuels for aviation and naphtha for the chemical industry. ArcelorMittal operates coal-based blast furnaces in Dunkirk.
Steel production is a massive source of carbon dioxide emissions, and the steel industry is under pressure to clean up its production. Technologies to do so are close to large-scale deployment. The most promising technology is hydrogen-based direct reduction of iron. ArcelorMittal is also experimenting with more experimental technologies that rely on purely electric steelmaking processes.
ArcelorMittal has received funding from the French government to decarbonize its operations in France. According to a company announcement from 2022, two of its three blast furnaces in Dunkirk should be replaced with hydrogen-based production by 2030. (ArcelorMittal already shut down one blast furnace in late 2022, so there are only two left by now.)
The two projects in Dunkirk - moving steel production away from coal and using steel's emissions to produce e-fuels and chemicals - appear to be in direct conflict. As long as steel plants are a source of carbon dioxide for e-fuels, they cannot be shut down and replaced with low-emission technologies.
"While other companies have announced to phase out coal-based blast furnaces, ArcelorMittal uses its 'Smart Carbon' pathway to stall effective climate action," says Caroline Ashley from SteelWatch, an environmental organization advocating for steel decarbonization. "Just last month, an ArcelorMittal representative told me point blank that they cannot rule out operating coal-based blast furnaces even in 2050. The demonstrated emissions reduction potential of their CCU projects is tiny compared to what is needed under EU climate targets."
ArcelorMittal did not answer a request to comment on its plans in Dunkirk. For the Reuze project, Malcolm Wells from Infinium commented: "In response to the EU compliance requirements for CO₂ by 2041, the Reuze partners are actively transitioning the venture to use biogenic CO₂ sources no later than 2041 to ensure compliant e-fuel production. This approach represents a compromise that will significantly reduce CO₂ emissions at the earliest opportunity, facilitating the commercialization of critical net-zero energy technology in Europe while avoiding the 'lock-in' scenario you have identified."
The EU compliance Infinium mentions refers to the EU regulation on recycled carbon fuels. It allows producing fuels from non-biogenic industrial CO₂ sources until 2040. It is one attempt by the European Union to find an answer to the question of CO₂ sources for CCU: Allow them for now, but move away from them in the future.
But even if Reuze transitions away from steel emissions by 2041, it raises questions. Will it delay moving ArcelorMittal's steel operations away from coal? How plausible is it to install a carbon capture unit at a steel plant that will only be operated for a short time period?
Both hydrogen-based steelmaking and the production of e-fuels will require vast amounts of clean hydrogen. Will there be enough for both in Dunkirk?
Carbon inputs and outputs
Looking at the bigger picture, we can view the question of CO₂ sources for CCU as one of carbon inputs and outputs. Climate change demands that we stabilize and eventually reduce the amount of carbon dioxide in the atmosphere.
For each carbon molecule extracted from the earth - whether from fossil fuels or carbon-containing minerals like in cement production - another carbon molecule needs to be stored permanently. Carbon molecules extracted from the atmosphere via biomass or direct air capture can be reused and enable CCU technologies. Some of those atmospheric carbon molecules should also go to permanent storage.
That means that CCU technologies with fossil or geological carbon emissions can, at best, be a temporary solution. They should have an exit strategy, and they should not delay decarbonization in other sectors.
This article was part one of a series about CCU. The second part (now online) covers the enormous energy requirements of CCU technologies, how thermodynamics makes things difficult - and why we may need to multiply the world's electricity production. A future newsletter will cover details about a CCU project at a cement plant. If you are not yet a subscriber, subscribe now so you won't miss it.
Author: Hanno Böck
I don't like paywalls, and I guess you don't like them either. You can read my articles for free, but researching and writing them takes time and effort.
If you enjoy reading this newsletter regularly, please consider supporting my work. You can donate to support my work via Patreon. If you work for a company or organization that may want to sponsor the newsletter or advertise in it, please get in touch.
If you do not want to support me financially, that is, of course, also fine. You can still help me tremendously by sharing this newsletter. If you found this text insightful, please forward it to others or share it on social media, in your organization's chat, or wherever you find appropriate.
Brief
-
Speaking of CCU: plans for an e-methanol plant in Antwerp, Belgium, have been scrapped due to escalating costs.
-
Where to use hydrogen and where to avoid it is a recurring and often controversial topic. The German town of Görlitz wants to use hydrogen to power a tram. John Worth calls it "about the worst idea I can imagine".
-
In Australia, steel company BlueScope will receive government funding to reinvest in its coal-based steelmaking facilities. SteelWatch points out that the money comes from a clean energy support program.
-
Regular readers will know about the problems with Europe's green electricity certificates (guarantees of origin). An interesting development to watch: The Irish advertising authority has reprimanded beer brewer Budweiser for claiming to use 100% renewable electricity, as those claims are based on guarantees of origin.